This reading is part of a series: Nuclear Waste Disposal
From the time it exits the reactor, operators watch over the nuclear waste like a hawk. They do this because the waste is intensely radioactive and highly dangerous. And it is precisely because of this that nuclear is, or rather should be, considered a clean energy source. Clean, not because it doesn't generate any waste, as every human activity creates waste – even the simple act of drinking water or breathing cannot escape this reality. But clean because we isolate its waste from the environment.
Relevant from the archive:
Controlling exposure to nuclear waste is challenging (link)
We strive to "leave no trace" in nuclear. Something we don't even aim for with most other waste we produce. We only think of isolating our waste if we think it could harm us down the line. It doesn't mean that our waste is not harmful to our environment. Plastic, for example, is widely used and is enormously helpful to our way of living. But its disposal or recycling is far from how it ought to be. It is in virtually everything we use. However, only 5% to 6% of plastic waste is recycled in the US [1]. Plastic that is not recycled can take thousands of years to decompose completely. Microplastics, which form when larger plastics in the environment break down, have been found almost everywhere, even in the Arctic, and in most sea creatures, from plankton to whales [2].
Plastic is but one example. How often do we really think about our waste? Many of us don't even care when throwing away a battery which contains toxic chemicals that last in the environment forever. But we would never dare to throw our waste from a nuclear reactor unless it was covered in impenetrable layers that isolate it from the environment. Imagine doing the same with CO2 from a fossil fuel energy plant or the tons and tons of waste we generate from other activities.
Hot, Hot, Hot!
Spent fuel from a nuclear reactor cannot be readily disposed of in a long-term geological repository when it exits the reactor. For one thing, it is extremely hot. Hot enough that it can melt by its own decay heat if not sufficiently cooled. The source of heat is the intense radioactive disintegrations in the spent fuel. All this makes it rather very complicated to handle.
Putting it into long-term storage right away is reckless for another reason. Heat tends to increase the rate of chemical reactions. Processes like corrosion of storage canisters and dissolution in groundwater tend to speed up. These are critical processes through which nuclear waste could travel from an underground storage site and pollute the environment before it decays to safe levels. Heat adds complexity to designing long-term storage.
Decay heat:
When the reactor stops, the spent fuel sitting idly in the reactor generates decay heat equal to about 6% of the power output of an operating reactor [3]. The fuel gets as hot as 1500oC when the reactor stops [4], hot enough to destroy the tubes that hold it. This is why the reactor continues to require cooling even when it stops. Loss of cooling can lead to a core meltdown, which happened at the Fukushima Daiichi nuclear power plant.
An hour after the reactor shuts down, the heat drops to 1.5% of the reactor output. In a week, it is 0.2% of the reactor output.
The first vessel for the storage of nuclear waste is the reactor itself. Fuel is allowed to cool down in the reactor for 5 to 10 days before being taken out.
After about a week, the spent fuel is transferred from the reactor to a large pool of cold water. After one year, the decay heat from the waste will be about 100 times less. In 30-40 years, it will be over 1000 times less [5].
The culprit:
The decay of fission products, especially Cs-137 and Sr-90, is responsible for the decay heat for the first 100 years. These two get so hot that a small truckload generates enough heat to power 200 homes [7]. Long-term decay heat is a result of Am-241 and Plutonium isotopes.
There are many economic and safety-related incentives to keep the waste close to the reactor without much handling and with easy access to cooling.
Cooling in a pool
The cooling pool or spent fuel pool deals with two immediate problems that nuclear waste creates: intense radioactivity and high decay heat.
Since the pool is right next to the reactor, it also reduces some of the complexity of safely handling radioactive material. The transfer of spent fuel from the reactor core to the pool and later from the pool to storage casks is all done underwater [8].
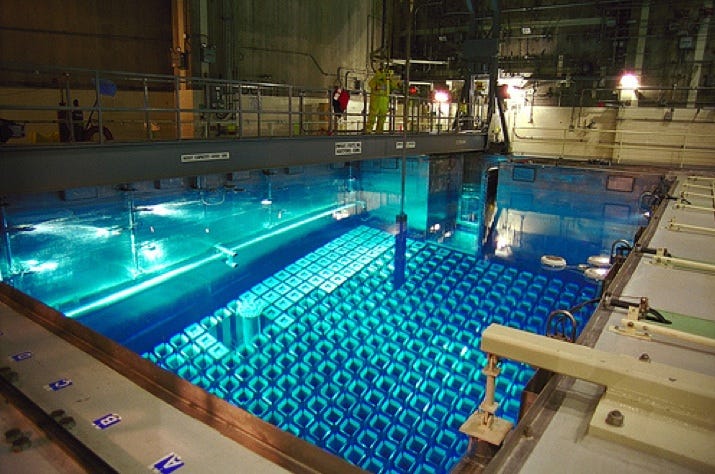
Pool structure and radiation shielding offered by water:
The cooling pool wall is made from thick steel-reinforced concrete with stainless steel liners and is often located below ground level [5].
The pool is around 40 feet deep and 40 feet in each horizontal dimension [8].
The bottom of the pool is where the assemblies to store the waste are kept. The storage racks are about 13 feet in height. Over twenty feet of water separates the waste from the surface. Water has a radiation absorption capacity of one-tenth that of lead. Twenty feet of protection is more than sufficient [8].
By wrapping around the waste, water acts as an excellent barrier, and as the spent fuel is very hot, water provides an ideal cooling solution to dissipate the decay heat from the waste. Apart from water, no additional shielding is required to contain the radiation.
The water in the pool is continuously monitored for radioactivity and filtered to ensure it is safe.
Water temperature must be controlled:
Water in the pool can itself get hot and requires constant cooling.
The total heat load of the cooling pool can be as high as 6MW when the spent fuel is first placed in the pool and falls to 4 MW ten days after [8].
Typically, one-third of the reactor core is emptied into the cooling pool at a time, but when the entire reactor core is emptied, the heat load of the cooling pool can be over 12 MW [8].
Active heat removal is vital for the safe operation of the cooling pool. The temperature is not allowed to rise above 60oC [8] and is usually much lower during normal operation.
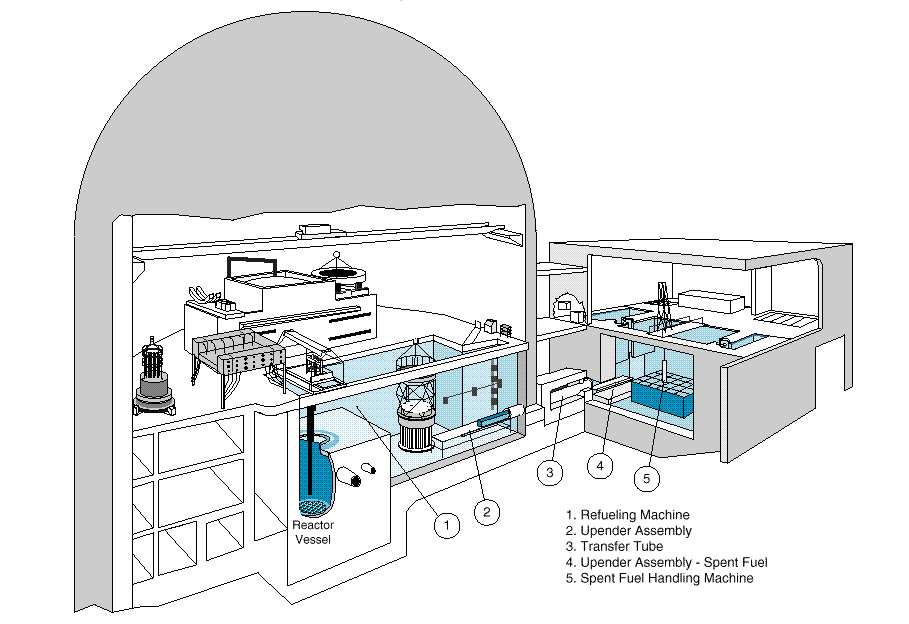
How long will it stay here?
After only a few years of sitting in the pool, the radioactivity and decay heat reduce considerably. Spent fuel is stored in a cooling pool for at least one year and typically for around 5 to 10 years.
After 2.5 years of cooling, the spent fuel has twice the decay heat per ton as spent fuel 5 years after discharge. The longer the spent fuel stays in the spent fuel pool, the cooler it gets and is less radioactive. Which means it gets easier to handle and store with time.
Dry casks in use today are typically licenced to accept spent fuel after 5 years of cooling. Dry casks can accommodate spent fuel earlier than 5 years. However, it would require substantial changes to the currently licenced casks in heat removal and radiation shielding [8].
Risks
How are they managed?
If, in the unlikely scenario, the cooling system of the spent fuel pool fails, the water will slowly boil off. If the water level drops enough to expose the tops of the fuel assemblies, the temperature of the assemblies will increase suddenly, accelerating the oxidation of the zirconium cladding that holds the spent fuel pellets. This can create vast amounts of heat and cause runaway failure of multiple assemblies. The failure of hot new fuel assemblies can lead to the failure of the cooler older fuel assemblies in the same cooling pool.
NRC deems the risk of such an event to be extremely small. Moreover, operators will have about 4 days to restore cooling before the water boils off enough to expose the top of the fuel assemblies [8]. However, if such an event were to occur, in the worst-case scenario, a large portion of radioactive material and gasses could be released into the environment.
The land contamination consequence of such an event could be significantly worse than that of the Chornobyl accident. In the Chornobyl accident, the release of cesium-137 (a very volatile radioactive constituent in a younger spent fuel) accounted for 2 megacuries of radiation. Given that a typical US spent fuel pool contains 400 tons of spent fuel, the total inventory of Cs-137 in the pool is 35 megacuries [9]. Between 10 to 100% of this Cs-137 could be released, which could cause tens of thousands of excess cancer deaths, loss of tens of thousands of square kilometres of land, and economic losses in the hundreds of billions of dollars [9].
Transfer out the older fuel:
There is good reason to transfer the older spent fuel out of the pool into dry cask storage, where active cooling is not required and where a failure of one cask is unlikely to propagate. An additional benefit of this is a reduction in the overall radioactive material inventory in the spent fuel pool, which would reduce the scale of the damage if it were to fail.
A point of view
The cooling system of the spent fuel pool is a single point of vulnerability. Loss of coolant has the potential, in the most extreme case, to release the entire inventory of radioactive material in the pool [8]. On the other hand, dry cask storage divides the vulnerability over many casks, where each failure would be local and containable. This alone makes the dry cask storage inherently safer. Dry casks have other advantages in terms of passive operation and low maintenance. As long as long-term geological storage remains unavailable, dry casks offer a superior solution for isolating spent fuel, especially for spent fuel older than 5 years, and maybe even sooner.
Explore other notes on nuclear waste
References:
[1] Faith Wakefield, “Top 25 recycling facts and statistics for 2022,” World Economic Forum, Jun. 22, 2022. Available: https://www.weforum.org/agenda/2022/06/recycling-global-statistics-facts-plastic-paper/.
[2] National Geographic Society, “Microplastics,” May 20, 2022. Available: https://education.nationalgeographic.org/resource/microplastics.
[3] MIT, “The Future of the Nuclear Fuel Cycle,” Massachusetts Institute of Technology, 2011.
[4] E. Kintisch, “Waste Panel Expected to Back Interim Storage,” Science, vol. 333, no. 6039, pp. 148–149, Jul. 2011, doi: 10.1126/science.333.6039.148.
[5] M. Nutt, “Spent Fuel,” Argonne National Laboratory, Mar. 04, 2016. Available: https://web.archive.org/web/20160304112040/http://www.anl.gov/sites/anl.gov/files/spent_fuel_nutt.pdf.
[6] A. Hedin, “Spent nuclear fuel - how dangerous is it? A report from the project ‘Description of risk,’” Swedish Nuclear Fuel and Waste Management Co., Stockholm (Sweden), 1997. Available: http://inis.iaea.org/search/search.aspx?orig_q=RN:29015601
[7] W. Cornwall, “Deep sleep,” Science, vol. 349, no. 6244, pp. 132–135, Jul. 2015, doi: 10.1126/science.349.6244.132.
[8] National Academy of Sciences, Safety and Security of Commercial Spent Nuclear Fuel Storage: Public Report. Washington, D.C.: National Academies Press, 2006. Available: http://www.nap.edu/catalog/11263.
[9] R. Alvarez et al., “Reducing the Hazards from Stored Spent Power-Reactor Fuel in the United States,” Science & Global Security, vol. 11, no. 1, pp. 1–51, 2003, doi: 10.1080/08929880309006.