Geological repository — a multi-barrier approach to ensuring long-term isolation of nuclear waste
the idea of multiple barriers | the various barriers employed | the roadblocks.
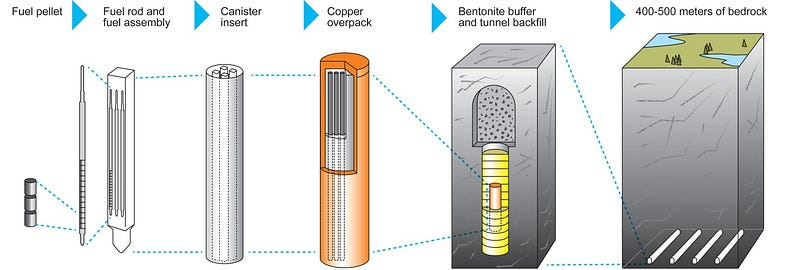
This reading is part of a series: Nuclear Waste Disposal
Geological repository designers bear an important responsibility. They must ensure that their design can never allow harmful nuclear waste to escape. They must ensure their design can survive for 100,000 years and work as intended throughout this time. They must ensure the unknowability of the future is overcome. They must also ensure their own limits do not jeopardize their mission. The designers feel this heavy burden. And if they perform their job well, their work will keep future generations safe. When the stakes are this high and there are so many unknowns, you build backups for the backups for the backups. You are cautious. You leave large margins. You are very conservative. And you build high levels of redundancy at each and every stage. The multi-barrier approach is such an approach, and it is employed in most repository designs.
A discussion on the mechanism through which harmful radioactivity can escape from a deep geological repository can be found here: (link)
Raisins in a muffin — the wasteform is the first barrier:
The spent nuclear fuel pellets are a complex amalgamation of several radionuclides that are constantly decaying. The radionuclides of concern are embedded in the fuel pellet like raisins in a muffin. The radionuclides are essentially trapped and isolated and can only be released if the spent fuel dissolves into the groundwater and reveals them. The wasteform, therefore, is the very first barrier.
Dissolution of the spent fuel pellet is a slow process. It would take longer than a million years to dissolve completely [1]. Consequently, if ideal conditions hold, dangerous radionuclides stay embedded in the waste without doing any harm and decay to harmless levels.
The fuel pellets are further encased in zirconium rods that are hard and highly corrosion-resistant.
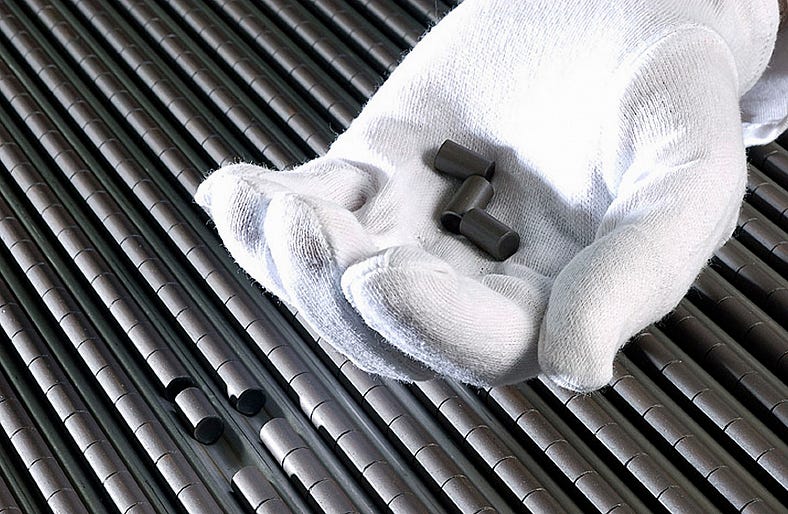
The canister encasing the waste:
Of course, ideal conditions may not hold. Zirconium cladding may crack. Groundwater flow may be higher than expected. The high heat from geological activity may speed up chemical processes like corrosion and dissolution.
For such contingencies and to delay water from reaching the radioactive waste, it is encased in an engineered canister. The canister is designed to work alongside the geology of the host rock to provide added protection, and its design is, for that reason, dependent on the type of geology chosen for the repository.
Onkalo repository canister:
The Onkalo repository, situated in crystalline bedrock, offers a reducing environment — an environment devoid of oxygen. Metallic copper has existed unchanged in such an environment for hundreds of millions of years [2].
Therefore, the canister chosen for this repository is a copper canister with iron inserts for structural support [3]. The iron helps maintain a reducing environment inside the canister. Actinides- the long-lived components of concern in nuclear waste- are much less soluble in groundwater in reducing environments.
The corrosion of the copper canister that would allow radionuclides to escape is supposed to take millions of years [4].
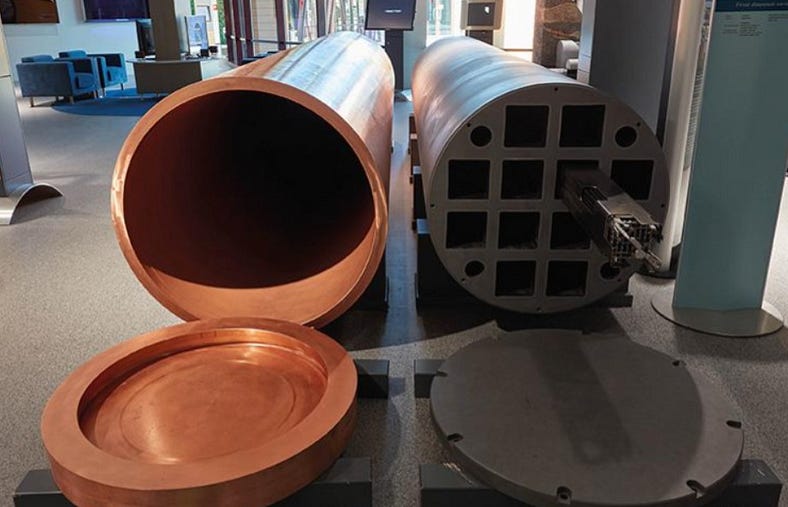
Yucca Mountain repository canister:
The Yucca Mountain repository, now canceled, was to be placed in consolidated volcanic ash above the water table in an oxidizing environment. The canister was to be constructed from corrosion-resistant nickel-based alloy with a titanium drip shield — a canopy to divert rainwater infiltrating the repository [5].
Although the arid region around Yucca Mountain receives very little precipitation and is sparsely inhabited — both ideal conditions for situating a geological repository, the geology’s oxidizing environment is unsuitable for the oxide fuel [6]. The spent fuel is much more soluble in this environment. Therefore, there was a much higher reliance on engineered barriers for assuring safety at Yucca Mountain. The expectation was that the region would remain arid and uninhabited, away from groundwater, reducing risk to future generations.
The clay around the canister:
For even more assurance and confidence in the design, the waste canister is surrounded by bentonite clay in some repository designs, like the Onkalo repository.
The clay buffer is a very effective transport barrier to long-lived radionuclides. Clay swells when it comes in contact with water and becomes almost impenetrable. The longer-lived radionuclides that manage to escape the canister will cling to the clay, thus slowing their movement considerably [4].
It is estimated that actinides (plutonium, americium, etc.) will take a few million years to travel through the buffer layer, by which time they would have long decayed to harmless levels [4].
The shorter-lived radionuclides like strontium and cesium can travel more easily through the clay buffer. The waste canister is expected to last much longer than it would take for these shorter-lived radionuclides to decay to harmless levels [4].
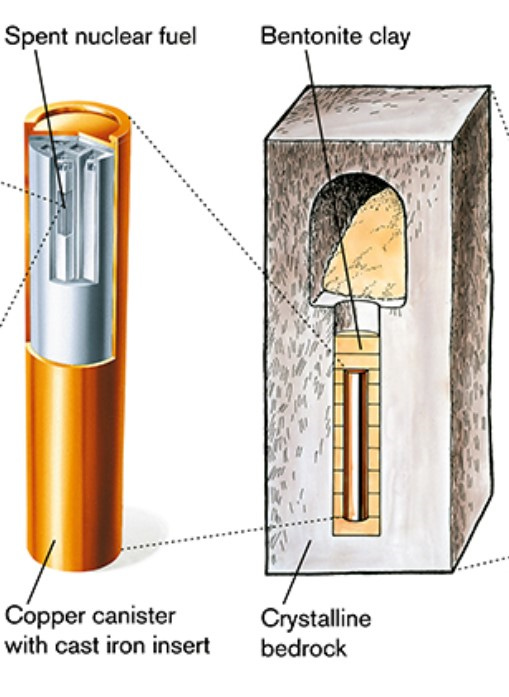
The rock around the clay:
If the engineered waste canister and the clay buffer should fail, the geology of the host rock acts as a barrier. Over the long term (greater than 10,000 years), engineered barriers cannot be necessarily relied upon, and the host site’s geology really provides long-term safety assurance [1].
Different approaches: rock, clay or salt:
A repository site is chosen such that the host rock’s geology remains stable over the foreseeable future. It should limit the mobility of groundwater and should be made of material that is sticky for the most dangerous radionuclides.
Several geologies offer these characteristics, and different countries are pursuing different options. The three categories being considered are high-strength crystalline rock (e.g. granite), sedimentary rock (e.g. clay), and salt. High-strength rock, like granite, has low porosity. Clay offers low permeability. There is no groundwater in salt geology, and its plasticity means any damaged canister gets sealed by the salt quickly [1].
Oxidizing vs. reducing environment:
The geochemical environment can be oxidizing or reducing depending on which geology is chosen. This affects the solubility of waste in groundwater.
Reducing conditions means the environment is oxygen-depleted. Repositories design being considered in granite and clay are in reducing conditions. Most radionuclides, but especially those considered dangerous over the long-term, like technetium-99 and neptunium-237 and other actinides, are orders of magnitude less soluble in groundwater in reducing environments [5]. Onkalo, the repository in Finland, is in deep granite under the water table in a reducing environment [8].
The selected sites have either no groundwater or very slow groundwater flow. Even with some groundwater flow, which itself could take several hundred to thousands of years to travel to the surface, the radionuclides would take thousands of times longer due to sorption on rock and matrix diffusion [4]. These processes turn the rock into a sticky and sponge-like material for the radionuclide. It’s like cleaning muddy water with a sponge, which turns muddy itself.
In oxidizing conditions, actinides are more soluble and mobile. Yucca Mountain repository was to be located in an oxidizing environment. The repository was situated above the water table, where flowing water is oxygen-rich. This was considered a significant drawback [9].
However, some scientists suggested that the unsaturated zone above the water table will remain essentially dry in desert areas. The very low precipitation in these areas will flow down through the rock cracks and mostly evaporate into the air [10]. Unlike in saturated areas, in unsaturated areas of desert regions, it would be easier to calculate the amount of water the waste comes in contact with [10]. In such a repository, the waste could be expected to remain nearly dry indefinitely [11].
Thus, a repository situated in an oxidizing environment relies on the assumption that the repository geology will remain dry for a very long time.
A point of view:
Despite the multi-barrier approach, not all repository designs have successfully convinced the public about their ability to safely isolate the waste. The roadblocks have almost always been not because of any technical difficulties but due to a lack of social engagement by repository designers.
Stakeholder participation from the start and a high level of inclusiveness have been a cornerstone of the nuclear waste program in Finland, where the Onkalo repository has been approved, and construction is already underway. The successful siting of the Waste Isolation Pilot Plant (WIPP) for transuranic radioactive waste that is operating in the US also had elements of inclusiveness. These were notably absent in the failed siting of the Yucca Mountain repository.
Stakeholder participation is about involving all relevant parties in the decision-making process. The idea behind it is that the people who would be most impacted by a project are usually not well informed, and therefore, an effort should be made to inform them and involve them in the decision-making from the beginning.
This ensures that values are incorporated into the decision-making process, which helps foster trust in the institutions.
Involving stakeholders helps take into account their concerns and expectations and provides access to their knowledge. It empowers the people affected, which allows them to gain confidence about the project and make informed decisions. This helps enable the adoption of more effective, sustainable, and fair decisions.
References:
[1] C. Corkhill and N. Hyatt, Nuclear Waste Management. IOP Publishing, 2018. [Online]. Available: http://iopscience.iop.org/book/978-0-7503-1638-5
[2] MIT, “The Future of the Nuclear Fuel Cycle,” Massachusetts Institute of Technology, 2011. [Online]. Available: https://energy.mit.edu/research/future-nuclear-fuel-cycle/
[3] Posiva, “Disposal canister.” Accessed: Dec. 12, 2023. [Online]. Available: https://www.posiva.fi/en/index/finaldisposal/releasebarriers/disposalcanister.html
[4] A. Hedin, “Spent nuclear fuel — how dangerous is it? A report from the project ‘Description of risk,’” Swedish Nuclear Fuel and Waste Management Co., Stockholm (Sweden), 1997. [Online]. Available: http://inis.iaea.org/search/search.aspx?orig_q=RN:29015601
[5] MIT, “The Future of Nuclear Power,” Massachusetts Institute of Technology, 2003.
[6] R. C. Ewing and F. N. von Hippel, “Nuclear Waste Management in the United States — Starting Over,” Science, vol. 325, no. 5937, pp. 151–152, Jul. 2009, doi: 10.1126/science.1174594.
[7] R. P. Rechard and M. D. Voegele, “Evolution of repository and waste package designs for Yucca Mountain disposal system for spent nuclear fuel and high-level radioactive waste,” Reliability Engineering & System Safety, vol. 122, pp. 53–73, 2014, doi: 10.1016/j.ress.2013.06.018.
[8] T. Vieno and H. Nordman, “Safety assessment of spent fuel disposal in Haestholmen, Kivetty, Olkiluoto and Romuvaara — TILA-99,” Posiva Oy, Finland, 1999.
[9] H. Feiveson, Z. Mian, M. V. Ramana, and F. von Hipple, “Spent Fuel from Nuclear Power Reactors: An Overview of a New Study,” International Panel on Fissile Materials, 2011. [Online]. Available: https://fissilematerials.org/library/ipfm-spent-fuel-overview-june-2011.pdf
[10] E. H. Roseboom, “Disposal of high-level nuclear waste above the water table in arid regions,” U.S. Geological Survey, 1983. [Online]. Available: https://www.osti.gov/servlets/purl/59660
[11] I. J. Winogard, “Radioactive Waste Disposal in Thick Unsaturated Zones,” Science, vol. 212, no. 4502, pp. 1457–1464, 1981, doi: 10.1126/science.212.4502.1457.