Modular Data Centers Meet Modular Energy
How Small Modular Reactors could transform data center power demand
Medium-Term Projections for Data Center Energy Demand
Data center demand, driven especially by AI growth, is set to surge over the next 20 years. In the near term, a compounded annual growth rate of 22% is projected for the next five years, with high-end estimates reaching up to 27% (source). This ambitious upper range assumes an unhindered pace of construction by tech companies.
In the past two years alone, annual investment in new data centers has doubled, with the majority of this surge occurring within the United States.
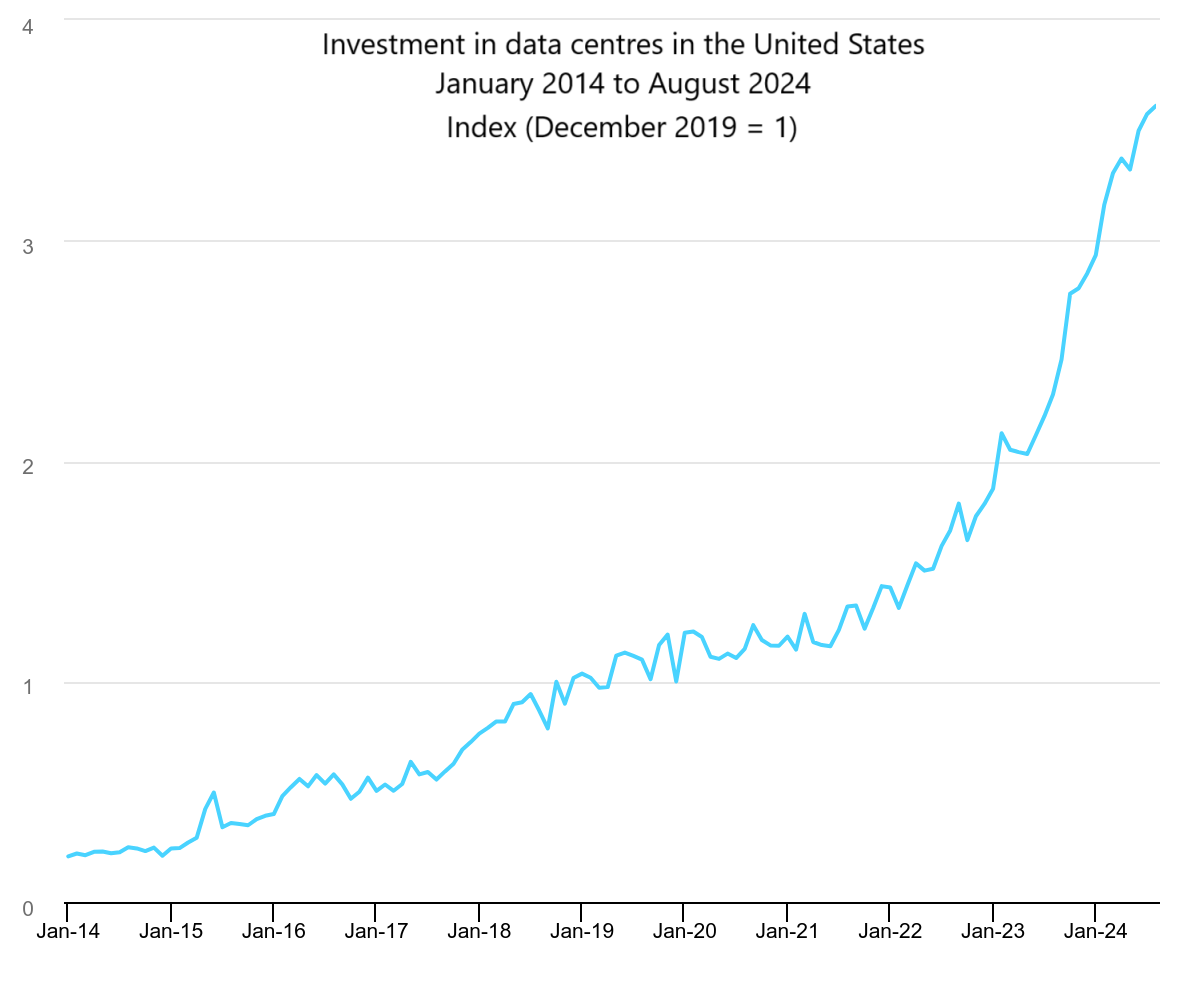
Data center infrastructure growth has accelerated sharply this year, with new announcements in the first half of 2024 tripling those from the same period last year and surpassing all of 2023's expansion (source). Yet significant barriers loom—not just in semiconductor availability, but in securing the colossal amounts of electricity these data centers require.
Energy Constraints as a Limiting Factor in AI and Data Center Growth
The growing demand for AI data centers could severely hinder progress toward net-zero goals. Already, data centers account for 2.5% of global CO₂ emissions—exceeding even the entire aviation sector (source).
While the full impact of AI on energy demand is likely to become evident only after 2030, AI data centers, though still a small segment, are expanding rapidly. Non-AI data centers' energy consumption is projected to climb from 142 TWh in 2023 to 304 TWh by 2030. In contrast, AI data centers are set to soar from 4 TWh in 2023 to 93 TWh by 2030—a more than 20-fold increase, according to Goldman Sachs (source). One can only imagine the strain on energy resources if this rate continues unabated.
Energy will ultimately be the critical bottleneck to AI’s expansion. To widen this bottleneck, AI companies will be willing to pay premiums for energy projects that once seemed unimaginable. Relying solely on the grid would be untenable as data center growth will soon create an enormous energy sink. Dedicated, co-located energy sources will be essential—not only because the grid cannot keep pace with such rapid expansion, but also due to the inefficiencies of relying solely on it.
Grid Capacity Challenges Amidst Rising Electricity Demand
The grid poses a major hurdle for AI companies. Surging demand from EV growth, widespread heat pump adoption, and the electrification of nearly all end-use energy is driving electricity demand at levels reminiscent of the 1960s, when the U.S. saw a boom in household appliances like refrigerators, air conditioners, dishwashers, and televisions. Over the next five years, electricity demand is expected to rise by 4-15% (source).
Insufficient growth of the grid also creates significant challenges for renewable energy, which relies on it to smooth out fluctuations in generation. Renewable growth has surged so quickly that grid limitations now actively hinder their broader adoption. Lawrence Berkeley National Lab estimates that nearly 2,500 GW of renewable electricity is waiting for grid connection, with delays stretching up to five years from construction to distribution (source).
If the grid continues to bottleneck growth—especially for energy-intensive businesses—on-site generation becomes an increasingly attractive solution. However, even with ample land adjacent to a factory or data center for solar or wind installations, supplemented by battery storage, seasonal shortfalls remain a challenge. On-site gas generators could also be used.
AI companies with a long-term strategic focus are beginning to recognize that securing their own energy sources may be essential for competitive survival. In a field where energy usage can determine operational limits, their future may hinge on this capability. AI queries are exceptionally energy-intensive—requiring 50 to 90 times the power of a typical Google search. More demanding processes, such as image generation, are even more costly: creating a single image consumes as much energy as charging over 500 smartphones (source).
Modular Data Centers Meet Modular Energy
Data centers are inherently modular, with components sourced from various specialized companies—chips from one, network components from another, storage solutions from yet another—all combining to form a scalable module. These modules can be linked to build clusters as large as needed. Recently, data center racks housed within shipping containers, which can be stacked and expanded on-site like bricks, have gained popularity. In essence, the only true connection these centers have to the outside world is through their cables—for data and electricity.
In many ways, Small Modular Reactors (SMRs) are designed with a similar philosophy. They consist of standardized, factory-produced components assembled and shipped directly to the client. Clients could request three units or thirty, scaling according to their energy requirements.
Positioning SMRs as an add-on component to a data center project seems ideal. The reactor units could be installed directly alongside or near the data center, tailored to meet specific energy needs. The cluster can grow by adding more modules, and as energy demands rise, additional SMRs can be incorporated to accommodate them.
Assessing SMRs for Enhanced Energy Autonomy and Resilience
Small Modular Reactors (SMRs) are significantly smaller than traditional nuclear reactors, typically under 300 MW and as low as 10 MW, compared to conventional reactors that often exceed 1,000 MW. Due to their compact size, SMRs can be located closer to populated areas, and their reduced scale makes them accessible to a broader range of customers.
SMRs are envisioned to be factory-built and transported to sites via conventional methods like trucks or trains. Factory construction enhances control over the manufacturing process, enabling the use of advanced techniques such as additive manufacturing and automation. This centralized approach allows greater quality assurance and consistency—imagine a "gigafactory" dedicated to producing these reactors.
Designed for mass production, SMRs offer cost savings through economies of serial production, akin to those in airplane and ship manufacturing. Here, cost efficiency stems from producing large quantities of identical units. After the initial reactors are constructed, subsequent units can be produced cheaply and more quickly than larger reactors, providing investors with greater confidence in timelines and risks. Like aircraft and ships, SMRs are modularly built and assembled into complete units (ideally, but not always) before delivery—a model intended to streamline production.
Proposed SMR designs are also simpler than today’s large reactors. Their lower total heat output means they often require only passive cooling systems, eliminating the complex plumbing needed for modern reactors, which reduces maintenance needs and enhances safety.
Some SMRs may also require less frequent refueling—certain designs are intended to operate using HALEU fuel (higher enrichment of U235) for up to 30 years without needing a fuel change (source).
Cost vs. Control
A common argument against SMRs and nuclear power is that they are too costly—much more so than renewables. Yet even at a premium, SMRs offer unique advantages. Chief among these is the autonomy and control they provide, reducing dependence on the grid. Higher costs may be justifiable if they mitigate vulnerabilities and guarantee reliable energy. For many major tech companies today, this assured availability is the driving factor in looking beyond renewables to nuclear. The added cost could, in fact, be seen as an investment in uninterrupted growth, ensuring that energy constraints do not hinder expansion.