Dry cask storage is a superior interim storage solution for nuclear waste
dry cask storage | passive systems.
This reading is part of a series: Nuclear Waste Disposal
Permanent disposal is not widely available for nuclear waste. Over the years, due to a lack of storage space, nuclear power plant operators have resorted to packing more and more spent fuel assemblies in the same spent fuel pool by placing them closer to each other. Dry cask storage was developed because they had no place left to store the spent fuel. Dry casks allowed them to expand on-site storage [1].
Relevant from the archive:
Nuclear waste fresh off the oven is piping hot (link)
When and for how long?
When is spent fuel transferred to dry casks?
Spent fuel is usually transferred to dry cask storage after at least 5 years of cooling in a spent fuel pool. However, it is possible to transfer the spent fuel earlier than that. After 2.5 years of cooling, the spent fuel has twice the decay heat per ton as spent fuel 5 years after discharge [2]. There are undoubtedly economic and safety-related incentives to store the fuel in the pool for longer than 5 years before transferring it [3]. For one thing, the longer the spent fuel stays in the spent fuel pool, the cooler it gets and is less radioactive. Which means it gets easier and safer to handle with time.
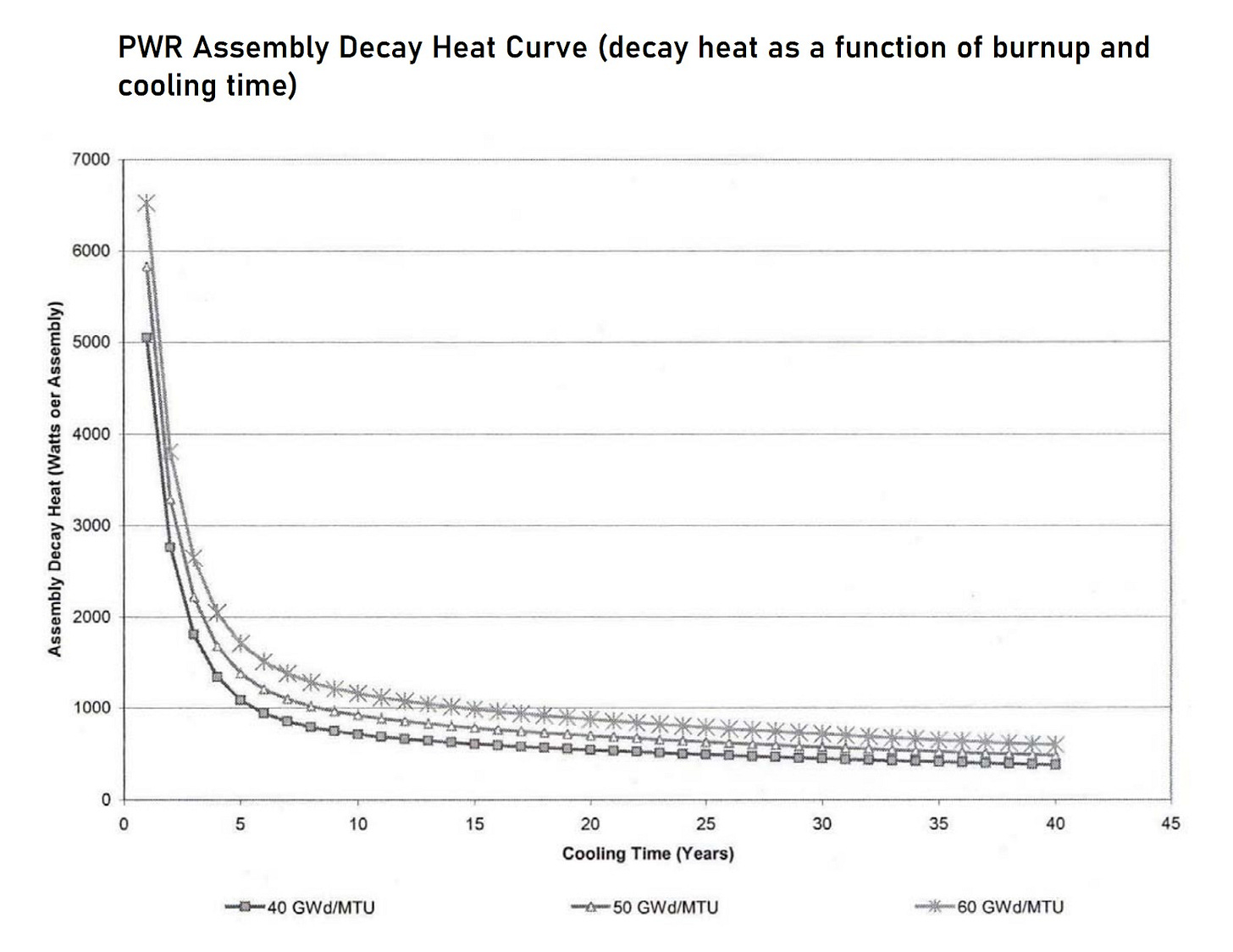
For how long can dry casks provide storage?
Dry cask storage can provide an interim solution for about 120 years [5] and perhaps longer. The hope is that a long-term solution will eventually be available to dispose of the waste.
For continued storage in dry casks for an extended period - lasting several centuries, replacement of older casks with newer ones will be necessary to prevent leaks, and continued maintenance will be required to ensure safety. Throughout this time, whether it’s a hundred or five hundred years, the facility must ensure sufficient funds are available for it to operate as intended - a risky proposition.
Structure and radiation shielding:
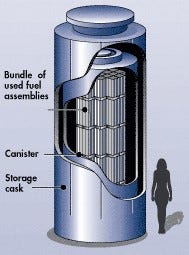
Each cask is typically 19 feet long and 8 feet in diameter. It can hold 10 to 15 metric tons of spent fuel and weigh 100 tons when fully loaded. Concrete, led, and steel are used to shield radiation and polyethylene, concrete and boron-impregnated metals are used to shield neutrons [1].
Dry casks have the advantage that they are robustly built and can take great punishment. Additional structures can be erected around them if additional shielding is required.
The concern:
Leaks can occur:
Dry casks can leak sometimes and have done so in the past. The failure of dry cask storage would be more benign and easier to manage than the failure of wet storage. A breach of a dry cask would release radioactive material through mechanical dispersion, a relatively slow process. It would result in a relatively small release of radioactivity near the cask itself. Any breach could be quickly plugged with radiation-absorbing material until a permanent fix or replacement could be done [1]. By contrast, spent fuel pool failure can contaminate a vast area.
Transferring spent fuel from one system to another is risky:
Transferring spent fuel from a spent fuel pool to a dry cask storage has its own set of hazards. During this process, the accident probability is higher than if the fuel is left in the pool [2]. This means that it was not worth the risk in the past if the spent fuel was to be transferred to geological storage in a few years anyway. However, given how slow the progress has been in developing a geological repository, dry cask storage will be used for several decades and maybe even for a century or more.
It may be safer overall to keep the density of spent fuel in the pool low and to transfer the spent fuel into dry casks as soon as it is viable.
It has been suggested that if the older fuel is removed from the pool and transferred to dry cask storage, and the new fuel in the pool is arranged in an open-rack configuration (providing plenty of space between fuel rods) and if large-scale airflow can be achieved in the building housing the pool, it could maintain spent fuel safely below its cladding failure temperature using convective air cooling alone [2].
Transferring older fuel not only assures that air cooling will become effective in a spent fuel pool in the event of a loss of cooling, reducing overall risk, but the reduced inventory of radioactive material will also sharply reduce the consequence of a failure. Removing older fuel will reduce the average inventory of Cs-137 in the pool by a factor of four [2].
This will reduce the overall risk of storing spent fuel.
Safety:
Passive Cooling:
Dry cask storage provides passive cooling – natural convection through the air is used to cool the spent fuel. Since there are no moving parts, little maintenance is required.
On the other hand, the spent fuel pool is an active system that requires constant monitoring. Loss of cooling can lead to accidents that release radioactive material.
Passive safety and its benefits:
A passive system relies only on natural forces or properties of the material that are known to be true beforehand, and that behave predictably due to the laws of nature [6].
These systems don't require external decisions or signals to work or operate. This means that an operator doesn't have to think about making the right decision, which avoids the consequences of making the wrong decision. The design of the system leads automatically to the desired action. Cooling happens automatically, without interruption and without the need for additional power or other input.
Passive systems are less complex and less susceptible to failure [7]. Failure in a passive system can still occur, but it is not a result of human error or power failure but due to mechanical or structural failure of components [8]. Dry casks can rupture and leak from radiation damage over time, but such failures are easily detectable and isolated, and the radioactive material only stays near the cask where it can be easily contained.
Passive systems can reduce the cost of maintenance associated with complex motor and pump setups usually associated with active systems [6].
Inherently safer:
Failure of a spent fuel pool would impact the entire stockpile of spent fuel in that storage. Dry cask's inherent advantage is not only in passive cooling but also in that it separates the fuel stored in one spent fuel pool into several discrete, robust containers. There is no single point of failure for the entire storage. If a failure occurs in the dry cask storage system, it happens in a smaller inventory of spent fuel, and the consequence of the failure is lower [1].
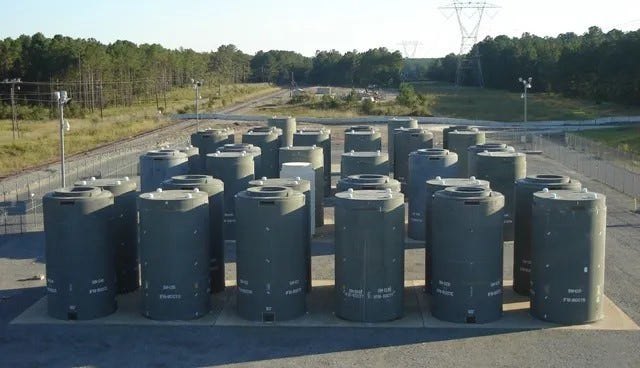
A point of view:
Future nuclear reactors using different and more advanced technology may not involve the pool storage phase. High-temperature gas-cooled reactors will use pebble bed fuel in which a tiny kernel of fuel is encapsulated in layers made of carbon and ceramic-based material that form a small pebble, which is highly resistant to high temperature and very robust [9]. Decay heat in waste from such reactors is 10 to 20 times less. The pebbles can survive harsh conditions for a million years and be directly disposed of in a geological repository [10]. This and other future technologies seek to make nuclear energy and waste management safer and more accessible.
Until a long-term solution becomes available, dry cask storage is critical to making the back end of the nuclear fuel cycle safer. It is important we don’t think of it as a permanent solution. It helps provide us time to evaluate the best long-term solution to nuclear waste.
Even though we call it waste, reactor technology currently in use only extracts a fraction of the energy in the fuel. Therefore, any long-term storage solution becomes complicated because permanent disposal that doesn't allow later retrieval of waste may not be the best option for the waste in its current form. And adding the option of retrievability can make the storage solution less than ideal.
References:
[1] National Academy of Sciences, Safety and Security of Commercial Spent Nuclear Fuel Storage: Public Report. Washington, D.C.: National Academies Press, 2006. [Online]. Available: http://www.nap.edu/catalog/11263
[2] R. Alvarez et al., “Reducing the Hazards from Stored Spent Power-Reactor Fuel in the United States,” Science & Global Security, vol. 11, no. 1, pp. 1–51, 2003, doi: 10.1080/08929880309006.
[3] MIT, “The Future of the Nuclear Fuel Cycle,” Massachusetts Institute of Technology, 2011. [Online]. Available: https://energy.mit.edu/research/future-nuclear-fuel-cycle/
[4] NRC, “Dry Cask Storage of Nuclear Spent Fuel,” 2010. [Online]. Available: https://www.sseb.org/downloads/Presentations/TRU/Easton.pdf
[5] H. Feiveson, Z. Mian, M. V. Ramana, and F. von Hipple, “Spent Fuel from Nuclear Power Reactors: An Overview of a New Study,” International Panel on Fissile Materials, 2011. [Online]. Available: https://fissilematerials.org/library/ipfm-spent-fuel-overview-june-2011.pdf
[6] IAEA, “Use of Passive Safety Features in Nuclear Power Plant Designs and their Safety Assessment.” [Online]. Available: https://www.iaea.org/topics/design-safety-nuclear-power-plants/passive-safety-features
[7] NEA, “Report on the Survey of the Regulatory Practice to Assess Passive Safety Systems Used in New Nuclear Power Plant Designs First Stage Report,” OECD Publishing, Paris, 2017. [Online]. Available: https://www.oecd-nea.org/jcms/pl_19816/report-on-the-survey-of-the-regulatory-practice-to-assess-passive-safety-systems-used-in-new-nuclear-power-plant-designs-first-stage-report?details=true
[8] IAEA, “Safety related terms for advanced nuclear plants,” 1991. [Online]. Available: https://www-pub.iaea.org/MTCD/Publications/PDF/te_626_web.pdf
[9] DOE Office of Nuclear Energy, “TRISO Particles: The Most Robust Nuclear Fuel on Earth,” Energy.gov, 2019. [Online]. Available: https://www.energy.gov/ne/articles/triso-particles-most-robust-nuclear-fuel-earth
[10] A. L. Lotts et al., “Options for treating high-temperature gas-cooled reactor fuel for repository disposal,” Oak Ridge National Laboratory, ORNL/TM-12077, 5350512, Feb. 1992. [Online]. Available: http://www.osti.gov/servlets/purl/5350512-fXloNS/