The Fiery Transformation: What Happens to Nuclear Fuel Inside a Reactor?
Inside a reactor, nuclear fuel takes a hell of a beating and becomes intensely radioactive.
This reading is part of a series: Nuclear Waste Disposal
Nuclear energy and fire - they are not so different
Uranium, in its natural form in the environment, is benign. In this form, it has existed since the beginning of our planet and will continue to be around long after we are gone. We have lived around it and over it without knowing what it is capable of for eons. And not even a hundred years have passed since we learned of the tremendous potential of this metal. The concept of flying is older than that.
The enormous power was its hidden secret, and it promised to propel our civilization into a future where the question of energy scarcity is no longer a concern. But this cannot happen if we don’t understand the complexity of dealing with it. The knowledge of the hidden secret of uranium has fundamentally altered our society and how our ‘war and peace’ is decided. We have come to fear it without questioning if the fear is justified. Complexity adds to the fear. We always fear what we don’t understand. Make no mistake, nuclear energy is inherently dangerous. But so is fire.
Fire and civilization
Is nuclear energy today not like the early days when we first discovered the awesomeness of fire? We must have seen the ferociousness of fire, how it destroys large swaths of land, engulfs entire villages, and burns without prejudice everything in its path to black ash. It is an uncontrollable force that nature unlashes when it gets too drunk with power. When it pleases to play with bright flames and wishes to see its thousand hands pillage the serene and the beautiful, the green, the brown, and the purple, red and blue.
Being “one with nature”, we would have, in the primordial days, already been witness to this form of energy and been afraid of it. And yet, there was something in this force of the gods that would lend a helping hand out from the hot flames and make our food taste better and easier to digest; that would shine light in the darkness and keep at bay the demons of the night from our loved ones. In time, we found a way to harness its deep powers to hunt, to forge things, and eventually, to build empires. We could have stayed away from this force, knowing it had widespread abilities to destroy and decide not to harness its hidden gifts. But that is not the human way. It is a monster that we befriended.
Nuclear energy and civilization
Nuclear energy today has many things similar to fire in those distant days. Our first introduction to it was of its destructive force. Its ability to annihilate. But those forces that give it this ability to inflict utter destruction are the same forces that could be harnessed to deliver potentially unlimited energy. And this energy comes in small packets of very high density.
Our per capita energy use is unlike our food or water consumption. We don’t drink more water or eat more food than our caveman days. But our energy use has multiplied exponentially. This requires that we pursue highly dense energy sources - coal has higher energy density than wood, and oil is denser than coal. And as our civilization expands, consumes more information, becomes more interconnected globally, grows on this planet and settles on other planets, our energy consumption will only increase. And that’s the way it should be too. Otherwise, innovation stops, progress stops, our exploration of new ideas, and the outer world stops. And are these not what makes us human?
Relevant from the archive:
The fuel takes a beating in releasing large quantities of energy.
Transmutation of nuclear fuel:
The environment inside a nuclear reactor is unforgiving. It gets hot. It is highly radioactive. And neutrons are flying around, hitting anything they find in their path. The fuel itself experiences significant restructuring and deformation directly through the nuclear reactions and indirectly through the thermo-mechanical conditions established by such reactions.
When U-235 or Pu-239, the fissile uranium and plutonium isotopes, fission, they form two smaller nuclei, more neutrons and energy. The smaller nuclei created are called fission products. Most fission products are short-lived and intensely radioactive. Two fission products, strontium-90 and cesium-137, with half-lives of about 30 years, account for the bulk of the radioactivity and decay heat from when the fuel leaves the reactor and for the initial few decades. Fission products dominate radioactivity in the spent fuel during the initial 100 years [1].
Sometimes uranium absorbs neutrons and doesn’t split. The new nuclei formed are heavier than uranium and are thus called transuranic elements, the most important being Pu-239. Neutron absorption and subsequent decay events results in the formation of elements like neptunium, plutonium, americium and curium. As a group, they are also called actinides. These actinides are long-lived and are responsible for most of the radioactivity in spent fuel after 500 years [1]. The half-life of most actinides is longer than the half-life of most fission products.
It is because of the actinides that long-term storage of nuclear fuel in a deep geological repository must be carefully thought out and engineered. Actinides have low solubility in groundwater and high sorption on rock. Repository designers use this fact while choosing the correct environment to place the repository. Some repository designs make it highly improbable for actinides to escape [3].
Physical transformation of nuclear fuel:
Apart from the intense radioactivity the fuel gains during its time in the reactor, it also becomes physically deformed. Remember in Iron Man 2 when Tony Stark pulls a brown, rusty, smoky, used-up palladium pellet out of his chest arc reactor? You can see the beating this pellet has taken. Something similar is happening to the uranium pellets in a nuclear reactor.
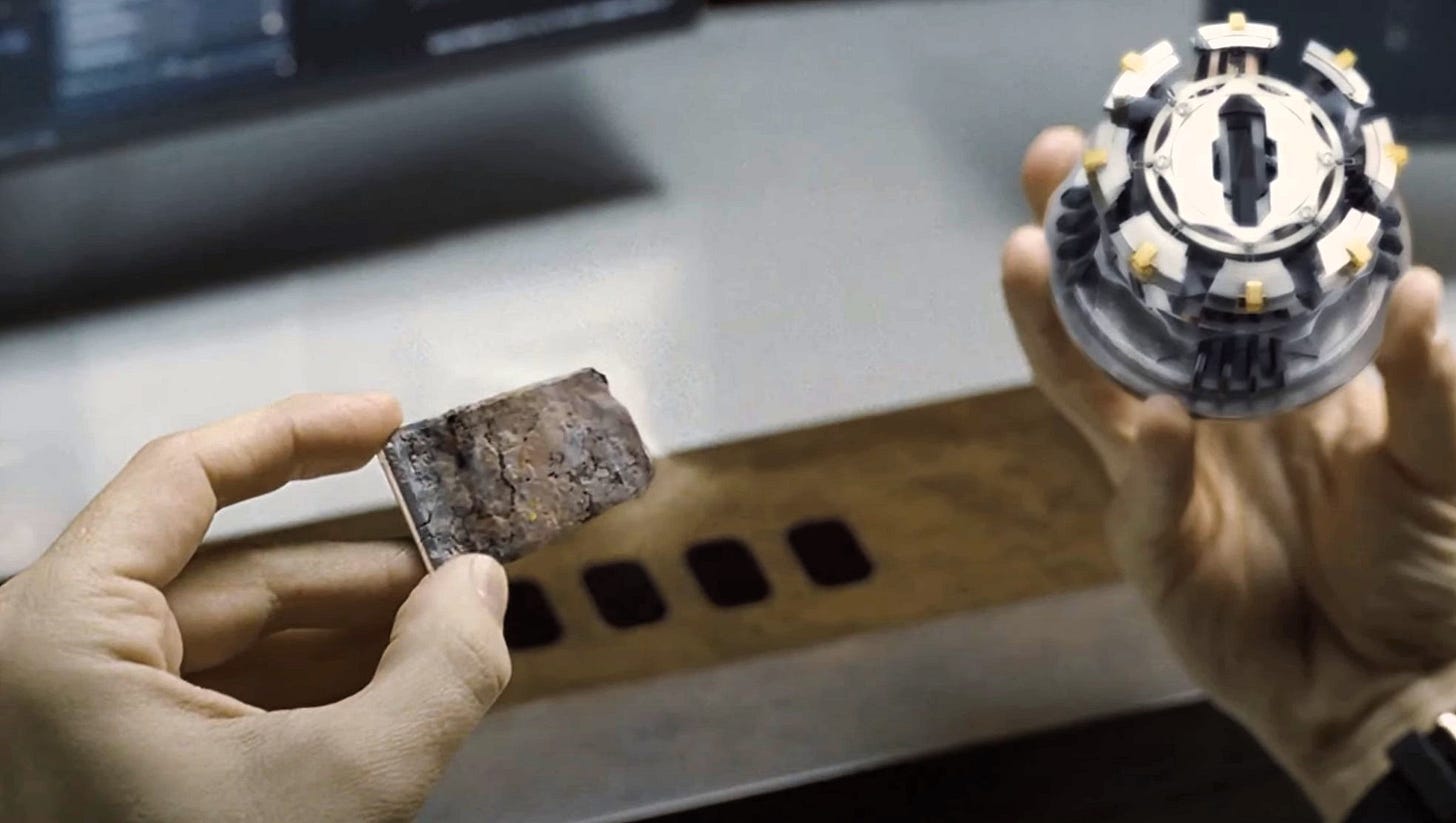
When uranium fissions, its energy is transferred to the surrounding fuel by the kinetic energy of fission fragments. The fission fragments travel as much as (~8 μm) within the fuel and knock off atoms during direct collisions, transferring their energy to those atoms, which can go and knock off other atoms in their path. This process generates heat that is then used for electricity generation. But a few years of constant beating can lead to permanent defects within the fuel. These defects can accumulate and grow. Apart from this physical change, there is a chemical change as uranium transmutes into new elements that the fuel structure must accommodate [2].
A high thermal gradient exists between the center and the surface of the fuel. The center of the fuel gets hot (~1700oC) compared to the outer rim (~400oC). The thermal stresses cause a network of cracks to develop in the fuel [4]. When fission products form as gasses (Xenon, Krypton) within the fuel, they can coalesce in the microcracks and form bubbles. Helium bubbles also form because of α-decay. Formation of these bubbles can cause the fuel to swell in volume.
Fuel rods are fabricated with a gap between the pellet and the zirconium cladding to accommodate swelling and deformation of the fuel pellet. The pellet-cladding interaction is a crucial point of vulnerability as the cladding is the first line of defence against radioactive fuel and prevents the fission gasses from releasing.
How long must the fuel endure this punishment?
After 3-4 years in a reactor, the fuel accumulates enough deformations and long-lived fission poisons (parasitic absorbers of neutrons) that it no longer efficiently produces electricity. When the reactor stops, the spent fuel is intensely radioactive and generates a large quantity of decay heat, equal to about 6% of the reactor's power output [3]. The decay heat decreases rapidly to 0.5% of reactor output within a week. The spent fuel is allowed to cool down in the reactor for about ten days, after which it is removed and put in a cooling pool next to it. It is kept there for at least 2-3 years.
Before entering the reactor, the fuel is only slightly radioactive and is handled without special shielding. In extracting energy from the fuel, the nuclear reactor fundamentally alters it. The formation of fission products, cesium-137 and strontium-90, is responsible for most of the high heat and radiation in the first 50 years after the fuel exits the reactor. Even after ten years of cooling, the spent fuel surface dose rate still exceeds 10,000 rem/hour (fatal whole-body dose for humans is about 500 rem received all at once). [5]
The act of taking raw ore out of the ground, converting it into fuel and extracting usable energy out of it, converts long-lived uranium into shorter-lived radioactive isotopes that result in a considerable increase in radioactivity for at least 100,000 years. This increase in radioactivity is the most apparent impact on the environment caused by nuclear energy generation. If we can safely isolate the highly radioactive spent fuel from the environment, then electricity generation from nuclear could be done sustainably [1].
[1] Hedin, A. (1997). Spent nuclear fuel - how dangerous is it? A report from the project 'Description of risk'. pp. 10,16,17. (SKB-TR--97-13). Sweden
[2] Rondinella, V. V., & Wiss, T. (2010). The high burn-up structure in nuclear fuel. Materials Today, 13(12), p.24. https://doi.org/10.1016/S1369-7021(10)70221-2
[3] MIT (2011). The Future of Nuclear Fuel Cycle: An Interdisciplinary MIT Study. pp 43,60
[4] Corkhill, C., & Hyatt, N. (2018). Nuclear Waste Management. pp. 4, 10. IOP Publishing. https://doi.org/10.1088/978-0-7503-1638-5
[5] Nuclear Regulatory Commission (2019, June). Backgrounder on Radioactive Waste. https://www.nrc.gov/reading-rm/doc-collections/fact-sheets/radwaste.html